MENÜ
CH | CHF
CH | CHF
Es konnten keine Ergebnisse gefunden werden.
Such-Empfehlungen
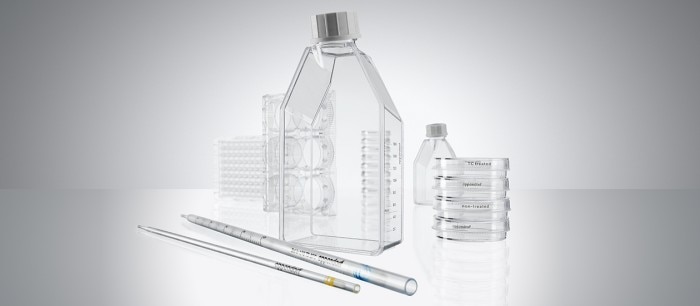
Cultureware: From Glass to Plastic
By William van Grunsven Lab Academy
- Bioprocessing
- Cell Biology
- Cell Culture
- Cell Culture Consumables
- Essay
Cell culture has come a long way since the establishment of the first cell lines in the 1950s, with improvements made in almost every aspect of the culture process. One of the key developments that affects scientists on a day-to-day basis has been the change from glass to plastic culture vessels.
Growing in glass
Glass has been the material of choice for biologists since long before the emergence of modern cell culture. When famous cell lines, such as HeLa and CHO , were established researchers had been growing tissue samples in glass vessels for many years [1]. So glass was the obvious choice for cell culture.
And why not? At first glance, glass seems to be the ideal material for growing cells. It’s a transparent, inexpensive material that is widely available. Glass is also resistant to a wide range of solvents, which seemingly made it easy to clean and reuse [2].
However, scientists soon noticed that clean glass surfaces are not the perfect material for cells to adhere to. Many cultured cells will only survive and proliferate when firmly anchored to their surroundings – as they would be inside the body, therefore coatings were often necessary to get efficient cell attachment and growth. However, applying and removing coatings added complexity and inconsistency to cell culture as these coatings can be hard to remove from the glass surface. Even when a cultureware surface looks clean to the naked eye, there can still be traces of biomolecules left from a previous experiment.
Moving on to plastic
As disposable plastics were becoming common in many aspects of daily life in the 1960s, they also became a mainstay of cell culture labs. Many different plastics have been used over the years, including polyethylene terephthalate (PET), high- and low-density polyethylene (PE), polyvinyl chloride (PVC) and polypropylene (PP) [3], but the material most frequently used in labs today is polystyrene (PS).
Polystyrene, used either as solid or a foam, is a ubiquitous material for applications such as packaging. In cell culture, its low material cost and inert chemistry make it well suited for use as a disposable culture surface, avoiding the need for cleaning and possible cross-contamination. Pure, solid polystyrene is also transparent, unlike the foams used for packaging, making it easy to observe cells in culture.
The low cost of polystyrene, combined with its low melting point compared to glass, also meant that cell culture vessels could now be produced in more complex shapes to suit an expanding range of cell culture methods. Vessels such as multi-well plates and specialized culture flasks had been developed earlier, but the move towards disposable vessels gave these products the lasting success that has continued to this day.
Improved surface properties
One of the key issues in developing polystyrene products for cell culture is getting the surface properties right. Cells adhere more readily to a hydrophilic surface, whereas pure polystyrene is hydrophobic. This issue is addressed by modification of the surface during manufacturing to improve the wettability of polystyrene [4]. The most common way of achieving this is by plasma oxidation. Plasma treatment partially modifies and cleaves the polymer chains, leaving behind acid and hydroxyl groups. This makes the surface more hydrophilic and improves the attachment of anchorage-dependent cells [1, 5].
The properties of polystyrene surfaces can be optimized further by coating the surface, for example using proteins, peptides or polysaccharides [6]. Protein coating is particularly useful when cells are grown under serum-free conditions, as serum is normally a good source of attachment proteins, such as fibronectin. Without this source of protein many cell types struggle to attach to even a tissue culture-treated (TC) surface [7].
Common proteins used for this purpose are those that are involved in cell attachment in the body. Collagen type I and IV, for example, are important structural proteins in many tissues and form good anchoring points for cells. Another popular choice is fibronectin, a glycoprotein that many cells produce to help with attachment. The surface of standard cell culture consumables can be coated with proteins following specialized protocols and by using coating media. Ready-to-use pre-coated cell culture consumables are also available.
Future developments
Plastic cell culture consumables are continuing to evolve as we further unravel the ways in which cells react to their environment. This search for optimal conditions often goes beyond surface chemistry; and the stiffness and texture of the surface are cited as being instrumental in driving proliferation and differentiation [8]. Also, we are learning more about the influence of different, often production-inherent, substances (e.g., slip agents, biocides, plasticizers, trace metals) on cell growth.
So, what of the next six decades of plastic cultureware development? As we discover more about plastics, and how cells interact with their environments, we will see ever-closer replication of the in vivo environment, while making complex cell culture more successful.
Glass has been the material of choice for biologists since long before the emergence of modern cell culture. When famous cell lines, such as HeLa and CHO , were established researchers had been growing tissue samples in glass vessels for many years [1]. So glass was the obvious choice for cell culture.
And why not? At first glance, glass seems to be the ideal material for growing cells. It’s a transparent, inexpensive material that is widely available. Glass is also resistant to a wide range of solvents, which seemingly made it easy to clean and reuse [2].
However, scientists soon noticed that clean glass surfaces are not the perfect material for cells to adhere to. Many cultured cells will only survive and proliferate when firmly anchored to their surroundings – as they would be inside the body, therefore coatings were often necessary to get efficient cell attachment and growth. However, applying and removing coatings added complexity and inconsistency to cell culture as these coatings can be hard to remove from the glass surface. Even when a cultureware surface looks clean to the naked eye, there can still be traces of biomolecules left from a previous experiment.
Moving on to plastic
As disposable plastics were becoming common in many aspects of daily life in the 1960s, they also became a mainstay of cell culture labs. Many different plastics have been used over the years, including polyethylene terephthalate (PET), high- and low-density polyethylene (PE), polyvinyl chloride (PVC) and polypropylene (PP) [3], but the material most frequently used in labs today is polystyrene (PS).
Polystyrene, used either as solid or a foam, is a ubiquitous material for applications such as packaging. In cell culture, its low material cost and inert chemistry make it well suited for use as a disposable culture surface, avoiding the need for cleaning and possible cross-contamination. Pure, solid polystyrene is also transparent, unlike the foams used for packaging, making it easy to observe cells in culture.
The low cost of polystyrene, combined with its low melting point compared to glass, also meant that cell culture vessels could now be produced in more complex shapes to suit an expanding range of cell culture methods. Vessels such as multi-well plates and specialized culture flasks had been developed earlier, but the move towards disposable vessels gave these products the lasting success that has continued to this day.
Improved surface properties
One of the key issues in developing polystyrene products for cell culture is getting the surface properties right. Cells adhere more readily to a hydrophilic surface, whereas pure polystyrene is hydrophobic. This issue is addressed by modification of the surface during manufacturing to improve the wettability of polystyrene [4]. The most common way of achieving this is by plasma oxidation. Plasma treatment partially modifies and cleaves the polymer chains, leaving behind acid and hydroxyl groups. This makes the surface more hydrophilic and improves the attachment of anchorage-dependent cells [1, 5].
The properties of polystyrene surfaces can be optimized further by coating the surface, for example using proteins, peptides or polysaccharides [6]. Protein coating is particularly useful when cells are grown under serum-free conditions, as serum is normally a good source of attachment proteins, such as fibronectin. Without this source of protein many cell types struggle to attach to even a tissue culture-treated (TC) surface [7].
Common proteins used for this purpose are those that are involved in cell attachment in the body. Collagen type I and IV, for example, are important structural proteins in many tissues and form good anchoring points for cells. Another popular choice is fibronectin, a glycoprotein that many cells produce to help with attachment. The surface of standard cell culture consumables can be coated with proteins following specialized protocols and by using coating media. Ready-to-use pre-coated cell culture consumables are also available.
Future developments
Plastic cell culture consumables are continuing to evolve as we further unravel the ways in which cells react to their environment. This search for optimal conditions often goes beyond surface chemistry; and the stiffness and texture of the surface are cited as being instrumental in driving proliferation and differentiation [8]. Also, we are learning more about the influence of different, often production-inherent, substances (e.g., slip agents, biocides, plasticizers, trace metals) on cell growth.
So, what of the next six decades of plastic cultureware development? As we discover more about plastics, and how cells interact with their environments, we will see ever-closer replication of the in vivo environment, while making complex cell culture more successful.
Mehr erfahren
Weniger lesen
References
1. Barker SL et al. Method of production and control of a commercial tissue culture surface. Journal of Tissue Culture Methods 1994;16: 151–153.
2. ATCC® Animal Cell Culture Guide. 2014.
3. Short R et al. Cell Culture Surface. 2005. Patent no. US20050244961A1.
4. Steele JG et al. Attachment of human bone cells to tissue culture polystyrene and to unmodified polystyrene: the effect of surface chemistry upon initial cell attachment. Journal of Biomaterials Science, Polymer Edition 1994;5(3): 245–257.
5. Zeiger AS et al. Why the dish makes a difference: Quantitative comparison of polystyrene culture surfaces. Acta Biomaterialia 2013;9: 7354–7361.
6. Qian L et al. Improving the expansion and neuronal differentiation of mesenchymal stem cells through culture surface modification. Biomaterials 2004;25: 1331–1337.
7. Mather JP et al. Introduction to Cell and Tissue Culture: Theory and Technique. Plenum Press, New York 1998.
8. Discher DE et al. Tissue Cells Feel and Respond to the Stiffness of Their Substrate. Science 2005;310(5751): 1139–1143.
1. Barker SL et al. Method of production and control of a commercial tissue culture surface. Journal of Tissue Culture Methods 1994;16: 151–153.
2. ATCC® Animal Cell Culture Guide. 2014.
3. Short R et al. Cell Culture Surface. 2005. Patent no. US20050244961A1.
4. Steele JG et al. Attachment of human bone cells to tissue culture polystyrene and to unmodified polystyrene: the effect of surface chemistry upon initial cell attachment. Journal of Biomaterials Science, Polymer Edition 1994;5(3): 245–257.
5. Zeiger AS et al. Why the dish makes a difference: Quantitative comparison of polystyrene culture surfaces. Acta Biomaterialia 2013;9: 7354–7361.
6. Qian L et al. Improving the expansion and neuronal differentiation of mesenchymal stem cells through culture surface modification. Biomaterials 2004;25: 1331–1337.
7. Mather JP et al. Introduction to Cell and Tissue Culture: Theory and Technique. Plenum Press, New York 1998.
8. Discher DE et al. Tissue Cells Feel and Respond to the Stiffness of Their Substrate. Science 2005;310(5751): 1139–1143.
Mehr erfahren
Weniger lesen
Related links
Mehr erfahren
Weniger lesen